Biochemistry - Comp Exam Flashcards
Compare activation and inactivation as methods of regulation.
Activation vs. Inactivation

Compare allosteric and competitive effectors in enzyme regulation.
Allosteric vs. Competitive Effectors

Compare covalent and noncovalent bonds.
Covalent Bonds vs. Noncovalent Bonds
- Covalent bonds:
- Strong
- Short
- Share electrons
- Noncovalent bonds:
- Weak
- Long
- Attraction only

Compare glycogen phosphorylase in liver and muscle.
Glycogen Phosphorylase - Liver vs. Muscle
- Liver and muscle forms of GP are isozymes, products of separate genes.
- Differ in their sensitivities to regulatory molecules.
- Liver enzyme:
- Inactivated by free glucose (indicator of blood sugar levels)
- Unaffected by AMP.
- Mutation in liver GP causes Hers disease.
- Muscle enzyme:
- Allosterically activated by AMP (measure of low energy status of cell).
- Mutation in muscle GP causes McArdle syndrome.
Compare glycolysis and gluconeogenesis.
Glycolysis vs Gluconeogenesis
- Glycolysis generates ATP, gluconeogenesis consumes ATP.
- Energy charge (ATP/ADP ratio) determines which pathway will be most active.
- Gluconeogenesis and glycolysis are reciprocally regulated.

Compare reversible and irreversible covalent modification in enzyme regulation.
Reversible vs. Irreversible Covalent Modification

Compare the 3 irreversible steps of glycolysis to the equivalent steps in gluconeogenesis.
Gluconeogenesis vs Glycolysis

Compare the kinetics of transporter-mediated diffusion to simple diffusion and channel-mediated transport.
Passive Transport Kinetics
- Straight line = zero-order.
- Curved line = first-order.

Describe a Lineweaver-Burk plot.
Lineweaver-Burk Plot
- Called a double reciprocal plot.
- Allows us to convert rates to a linear graph.

Describe ABC transporters.
ATP-Binding Cassette Transporters
- 150 ABC transporter genes in human genome.
- Mechanism:
- The catalytic cycle begins with the transporter free of both ATP and substrate. While the distance between the ATP-binding cassettes in this form may vary with the individual transporter, the substrate binding region of the transporter faces inward.
- Substrate enters the central cavity of the transporter from inside the cell. Substrate binding induces conformational changes in the ATP-binding cassettes that increase their affinity for ATP.
- ATP binds to the ATP-binding cassettes, changing their conformations so that the two domains interact strongly with one another. The close interaction of the ABCs reorients the transmembrane helices such that the substrate binding site is now facing outside the cell.
- The outward facing conformation of the transporter has reduced affinity for the substrate, enabling the release of the substrate on the opposite face of the membrane.
- The hydrolysis of ATP and the release of ADP and inorganic phosphate reset the transporter for another cycle.
- Example - multi-drug resistance.
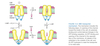
Describe allosteric regulation of glycogen phosphorylase (GP) in the liver.
Glycogen Phosphorylase - Allosteric Regulation in Liver
- Default “a” form or active form.
-
Inactivated by glucose:
- Glucose binds to active site and stabilizes conformation in the inactive T state.
- When glucose levels high, no need for glycogen breakdown (which will make more glucose).

Describe allosteric regulation of glycogen phosphorylase (GP) in the muscle.
Glycogen Phosphorylase - Allosteric Regulation in Muscle
- Default “b” form or inactive form.
-
Activated by AMP.
- Binds to active site and stabilizes conformation of b in the active R state.
- During muscle contraction ATP converted to AMP by myosin and adenylate kinase signaling the GP to breakdown glycogen.
-
ATP and Gluc-6-phosphate are negative allosteric regulators.
- Under normal physiological conditions GP inactive because of inhibitory effect of ATP and Gluc-6-phosphate.

Describe allostery.
Allostery
- Binding that does not occur at the active site.
- Heteroallostery - modulator and substrate are different.
- Homoallostery - modulator and substrate are the same.
Describe aspartate transcarbamoylase (ATCase).
De Novo Pyrimidine Synthesis - Aspartate Transcarbamoylase (ATCase)
- Catalyzes reaction between carbamoyl phosphate and aspartate to form carbamoylaspartate.
- 2 allosteric regulators:
- ATP activates.
- CTP inhibits.

Describe base pairing in nucleic acids.
Nucleic Acids - Base Pairing
- Purines always base-pair with pyrimidines, which creates an antiparallel double helix.

Describe biochemical strategies to drive an unfavorable reaction.
Biochemical Strategies for Driving Unfavorable Reactions
- Maintain Q < K
- Example: create a pathway to use up the products.
- Couple reaction to a highly favorable reaction.
- Example: ATP hydrolysis.
- ΔG values can be summed.
Describe branch transfer and release of glucose in glycogenolysis.
Glycogenolysis - Branch Transfer and Release of Glucose
- Transferase transfers a block of 3 of the remaining 4 glucose to the non-reducing end of the main chain forming an α-1,4 bond.
- Debranching enzyme or α-1,6 glucosidase cleaves the α-1,6 bond of the single remaining glucose residue to release the free glucose. Glucose phosphorylated by hexokinase.
- Transferase and α-1,6 glucosidase convert branched glycogen into a linear structure for further action by GP.

Describe carbamoyl phosphate synthetase II (CPSII).
Carbamoyl Phosphate Synthetase II (CPSII)
- First step in pyrimidine synthesis.
- Has a channel, through which the intermediates travel.
- Active sites are called ATP-grasp folds, which surrounds and holds ATP in position for a nucleophilic attack at the γ phosphoryl group.
- Net of 3 reactions.
- Requires 2 ATP.
- Uses glutamine as amine donor.

Describe catabolism of nucleotides.
Catabolism of Nucleotides
- Purines:
- Final product of catabolism is Uric acid.
- Pyrimidines:
- Final product of catabolism is β-Ureidopropionic acid.
- β-Ureidopropionic acid can be converted to alanine + CO2 + NH3 by the enzyme Ureidopropionase to complete pyrimidine catabolism.
- Final product of catabolism is β-Ureidopropionic acid.

Describe competitive inhibition.
Inhibition - Competitive
- Competing with S for active site.
- Lineweaver-Burk plots for rate with and without inhibitor, cross at y-intercept.
- νmax is constant.
- Km is variable.

Describe complex I of the ETC.
ETC - Complex I
- Complex I aka NADH dehydrogenase aka NADH-Q oxidoreductase.
- Large protein (>900 kDa, with 46 polypeptide chains).
- Encoded by both nuclear and mitochondrial genes.
- First point of entry of electrons from NADH.

Describe complex II of the ETC.
ETC - Complex II
- FADH2 enters the ETC through Succinate-Q reductase complex (Complex II).
- This complex connects TCA to Oxphos (Succinate Dehydrogenase).
- FADH2 does not leave the complex.
- Its electrons transferred to FeS and then to Q to form QH2.
- Does not pump protons.
- Consequently less ATP synthesized from oxidation of FADH2.

Describe complex III of the ETC.
ETC - Complex III
- Electrons from QH2 are passed on to cytochrome c by cytochrome c oxidoreductase aka Complex III.
- Flow of electrons through this complex leads to transport of 2 protons to cytoplasmic side.

Describe complex IV of the ETC.
ETC - Complex IV
- Last complex aka Complex IV.
- Cyt c oxidase catalyzes transfer of electrons from reduced Cyt c to molecular oxygen, the final acceptor.
- Makes these reactions aerobic.
- Makes humans “breathe”.
- Four electrons funneled to oxygen to reduce it to water.
- Concomitantly protons are pumped from matrix to cytoplasmic side of inner membrane.

Describe configurational isomers.
Isomers - Configurational
- Have chiral Cs.
- 2 types:
- Diastereomers - isomers, with multiple chiral centers, that are not mirror images of each other.
- Enantiomers - isomers, with a single asymmetrical C, that are mirror images of each other.
- Example:
- D -glyceraldehyde and L -glyceraldehyde.
- Example:

Describe conformational isomers.
Isomers - Conformational
- Reversible rotational changes.
- Pyranose rings (6C):
- 2 types:
- Chair
- Substituents on the ring carbon atoms have two orientations:
- Axial - bonds are nearly perpendicular to the average plane of the ring.
- Substituents can sterically hinder each other if they emerge on the same side of the ring
- Equatorial - bonds are nearly parallel to this plane.
- Substituents are less crowded.
- Axial - bonds are nearly perpendicular to the average plane of the ring.
- Substituents on the ring carbon atoms have two orientations:
- Boat
- Chair
- Chair form of β-D-glucopyranose predominates because all axial positions are occupied by hydrogen atoms. The bulkier -OH and -CH2OH groups emerge at the less-hindered periphery.
- Boat form of glucose is disfavored because it is quite sterically hindered.
- 2 types:
- Furanose rings (5C):
- Pucker - 4 atoms nearly coplanar with 1 atom ~0.5 A out of plane.
- 2 conformations:
- C-2-endo - C2 is out of plane on the same side as C5.
- C-3-endo - C3 is out of plane on the same side as C5.

Describe constitutional isomers.
Isomers - Constitutional
- Order of atoms changes.
- Called tautomers:

Describe conversion from Fisher to Haworth projections.
Converting Fisher to Haworth
- Determine 5 or 6 C ring.
- Carbonyl C goes 1 position CW from O.
- Number Cs CW.
- The =O becomes OH:
- α - OH down.
- β - OH up.
- L side of Fisher goes up, R side goes down.
- Until you reach last C before O in the ring.
- Remaining Cs are rotationally ambiguous.

Describe cooperativity.
Enzymes - Cooperativity
- Binding of each subsequent ligand influences the affinity (strength of interaction) of the next ligand to bind an active site.
Describe cooperativity.
Cooperativity
- Binding of an O2 molecule to heme facilitates the binding of O2 to another heme.
- Conformational change in one globin subunit induces a conformational change in another subunit.
- Causing increase in affinity with each successive O2 molecule that binds.
- Also applies to release of O2 in tissues.
- Each successive loss of an O2 molecule decreases affinity.
- Cooperativity is what makes Hb a good O2 carrier.
- 2 models:
- Sequential model:
- Each subunit of hemoglobin exists in T or R state.
- Each subunit that binds O2, causes the adjacent subunit to change from T to R sequentially.
- Concerted model:
- All subunits exist in T or R state.
- Strongly favors T state when no O2 bound.
- Strongly favors R state when O2 is bound.
- Sequential model:

Describe cysteine’s ability to form a disulfide bond.
Cysteine
- Only AA capable of making disulfide bond through a redox reaction.
- All proteins with disulfide bonds must have at least 2 cysteines.

Describe de novo synthesis of purines.
De Novo Synthesis of Purines
- Starts with PRPP and forms the nitrogenous base on the ribose ring, as opposed to pyrimidine synthesis where the nitrogenous base is formed first and then added to PRPP.
- Branched pathway, from inosine monophosphate (IMP) we can either make GMP or AMP.
- Cytoplasmic
- Precursors:
- NH3 from Gln.
- Gly, Asp
- N10-formyl-THF (Vitamin B9)
- HCO3-
- Regulation:
- Feedback by purines.
- Reactions:
- 1-3 - form the smaller, 5-member ring:
- Swaps PPi on PRPP for NH3 from Gln.
- Adds glycine.
- Adds a formyl group from N10-formyl THF to complete the ring.
- 4-10 form the 6-member ring:
- Adds NH3 from Gln to start the second ring.
- Closes the 5-member ring.
- Adds CO2 from HCO3- first to Gln (N) then to Gly (α-C).
- Adds Asp at the carboxyl.
- Releases Fumarate.
- Second addition of a formyl group from (another) N10-formyl THF (vitamin B9), completing the 6-membered ring.
- Closes the 6-membered ring, forming hypoxanthine.
- 1-3 - form the smaller, 5-member ring:
- Double ring structure is hypoxanthine, entire nucleotide is called inosinate (IMP).
- Reactions 1-10 occur in one enzyme complex consisting of 6 enzymes.
- 1-4 occur in individual proteins.
- 2,3,5; 6,7; 9,10 carried out on 3 mutlifunctional proteins.
- Carried out in close proximity so intermediates can easily be passed from domain to domain.
- 2 additional enzymes provide the N10-formyl-THF.
- IMP can then make either AMP or GMP.
- AMP and GMP get converted through two kinases to ATP and GTP.

Describe de novo synthesis of pyrimidines.
Pyrimidines - De Novo Synthesis
- Unidirectional pathway.
- Cytoplasmic.
- Precursors:
- -NH3 from Gln.
- Asp
- HCO3-
- Allosteric Regulation:
- Pyr inhibits (C)
- Pur activates (A/G)
- Ring is synthesized first then attached to a ribose phosphate to form a pyrimidine nucleotide.
- Assembled from bicarb, aspartate, and NH3.
- NH3 may already be present in solution but usually obtained from glutamine.
- Reactions:
- 6 reactions to form pyrimidine:
- CAD - multifunctional, eukaryotic protein performs the functions of:
- Carbamoyl phosphate synthetase II (CPSII).
- Aspartate transcarbamoylase (ATCase).
- 2 allosteric regulators:
- ATP activates.
- CTP inhibits.
- 2 allosteric regulators:
- Dihydroorotase
- Dihydroorotase dehydrogenase.
- Located in mitochondria.
- UMP synthetase:
- Multifunctional protein, performs two functions.
- Nucleoside monophosphate kinases.
- Specific to each NMP
- Nucleoside Diphosphate Kinase.
- Broad specificity.
- CTP synthetase
- 2 allosteric regulators just like ATCase:
- CTP inhibits.
- GTP activates.
- 2 allosteric regulators just like ATCase:
- CAD - multifunctional, eukaryotic protein performs the functions of:
- 6 reactions to form pyrimidine:

Describe diastereomers.
Isomers - Diastereomers
- 2 types:
- Epimers - diastereoisomers differing in configuration at only a single asymmetric center.
- Note that D-glucose and D-mannose differ in configuration only at C-2, the carbon atom in the second position. Thus, D-glucose and D-mannose are epimeric at C-2; D-glucose and D-galactose are epimeric at C-4.
- Anomers - when carbohydrates form a cyclic hemiacetal (ring), creating an additional asymmetric center.
- In glucose, C-1 (the carbonyl carbon atom in the open-chain form) becomes an asymmetric center (anomeric C). Thus, two ring structures can be formed:
- α-D-glucopyranose and β-D-glucopyranose.
- α designation means that the hydroxyl group attached to C-1 is on the opposite side of the ring as C-6.
- β designation means that the hydroxyl group is on the same side of the ring as C-6.
- In glucose, C-1 (the carbonyl carbon atom in the open-chain form) becomes an asymmetric center (anomeric C). Thus, two ring structures can be formed:
- Epimers - diastereoisomers differing in configuration at only a single asymmetric center.
- Monosaccharides made up of more than three carbon atoms have multiple asymmetric carbons, and so they can exist not only as enantiomers but also as diastereoisomers.
- The number of possible stereoisomers equals 2n, where n is the number of asymmetric carbon atoms.
- Six-carbon aldose with 4 asymmetric carbon atoms can exist as 16 possible diastereoisomers, of which glucose is one such isomer.

Describe dihydroorotase.
De Novo Pyrimidine Synthesis - Dihydooritase
- Assists carbamoylaspartate to cyclize forming dihydroorotate.
- Dihydroorotate is oxidized by NAD+ to form orotate.
- Opposite of hydrolase.

Describe dihydroorotate dehydrogenase.
De Novo Pyrimidine Synthesis - Dihydroorotate Dehydrogenase
- Located in inner membrane of mitochondria.
- Converts dyhydroorotate to orotate by removing water and forming double bond.
- Uses FMN to accept e- forming FMNH2.
- FMNH2 passes e- to coenzyme Q in ETC forming QH2.

Describe dipole electrostatic interactions.
Electrostatic Interactions - Dipoles
- Dipole - means having a partial charge.
- Permanent (polar)
- Induced (polarizable)

Describe disaccharides.
Disaccharides
- Consists of two sugars joined by an O-glycosidic bond.
- Name gives all info.
- α - hydroxyl points down.
- β - hydroxyl points up.
- Three common disaccharides:
- Sucrose (common table sugar):
- Obtained commercially from sugar cane or sugar beets.
- Anomeric carbon atoms of a glucose unit and a fructose unit are joined in this disaccharide.
- Configuration is α for glucose and β for fructose.
- Can be cleaved into its component monosaccharides by the enzyme sucrase.
- Lactose:
- Consists of galactose joined to glucose by a β-1,4-glycosidic linkage.
- Hydrolyzed to these monosaccharides by lactase in human beings and by β-galactosidase in bacteria.
- Maltose
- Consists of two glucose units joined by an α-1,4-glycosidic linkage.
- Comes from the hydrolysis of large polymeric oligosaccharides such as starch and glycogen.
- Hydrolyzed to glucose by maltase.
- Sucrose (common table sugar):
- Sucrase, lactase, and maltase are located on the outer surfaces of epithelial cells lining the small intestine.
- The cleavage products of sucrose, lactose, and maltose can be further processed to provide energy in the form of ATP.

Describe dispersion (van der Waals forces) electrostatic interactions.
Electrostatic Interactions - Dispersion

Describe enzyme inhibitors.
Enzymes - Inhibitors
- Can be:
- Irreversible
- Reversible
- Reversible:
- Use noncovalent interactions to bind.
- 3 types:
- Competitive
- Noncompetitive (allosteric)
- Uncompetitive (allosteric)
Describe equilibrium.
Equilibrium
- Dynamic equilibrium - making and/or breaking chemical bonds.
- Most reactions are reversible.
- Does not mean equal concentrations.

Describe essential vs non-essential amino acids.
Amino Acids - Essential vs Non-Essential
- Essential - must consume, cannot be synthesized by the body.
- 2 exceptions - argenine and methionine can be synthesized, but are considered essential because consuption > production.
- Non-essential - body can synthesize.
- 1 exception - tyrosine can be made through secondary synthesis by adding an OH to phenylalanine, however it is only non-essential as long as there is an adequate supply of phenylalanine.

Describe FA translocation to mt matrix.
Fatty Acids - Translocation to mt Matrix

Describe facilitated diffusion.
Facilitated Diffusion - Ion Channels
- 2 important features:
- Selectivity filter:
- Varies based on ion allowed to pass.
- Example K+:
- Although Na+ is smaller than K+, K+ channels are 100x more permeable to K+.
- Due to O atoms in selectivity filter.
- Make the dehydration of K+ more favorable than the dehydration of Na+.
- Gate (three ways):
- Voltage
- Ligand
- Stress
- Selectivity filter:
- Bacterial K+ channel:
- Movement results from electrostatic repulsion.

Describe fatty acid synthase (FAS).
Fatty Acid Synthase (FAS)
- Large multi-enzyme complex.
- Composed of 2 identical dimers (260 kDa each).
- Two dimers arranged in head to tail conformation.
- Each has 7 enzyme activities and an acyl carrier protein (ACP).

Describe fucose.
Fucose
- Fucose is wierd.
- Usually when we replace the -OH we are left with an O or N except in the case of fucose.
- Galactose derivative
- Know if there is a methyl attached to C5 that it is fucose.
- Only L-monosaccharide made and used by mammals.
- Part of A/B/O blood antigens.
- Excess free fucose in blood = liver damage, cancer, diabetes, heart disease.

Describe G6PD regulation.
Glucose 6-Phosphate Dehydrogenase - Regulation
- Regulation by:
- Transcription/Translation control.
- Location in cell.
- Post-translational controls.
- Activators:
- Dimerization
- Transcription factors for antioxidant genes.
- Cell cycle and synthesis activators.
- Insulin
- Inhibitors:
- Phosphorylation
- Apoptosis-signaling proteins.

Describe G6PD.
Glucose 6-Phosphate Dehydrogenase
- First step in the oxidative phase of PPP.
- Catalyzes the dehydrogenation of G6P to 6-phosphoglucono-δ-lactone.
- Essentially irreversible.
- Rate limiting.
- 3 forms:
- Monomer
- Dimer
- Tetramer.
- NADPH functions as both a substrate and a coenzyme.

Describe Gibbs free energy.
Gibbs Free Energy

Describe gluconeogenesis.
Gluconeogenesis
- Occurs in liver and kidney.
- Synthesis of glucose from non-carbohydrate precursors.
- Pathway not a reversal of glycolysis.
- Pathway converts pyruvate into glucose.
- Major precursors are lactate, amino acids, and glycerol.
- “By-passes” the irreversible steps of glycolysis through four enzymes not present in glycolysis:
- Pyruvate carboxylase
- Phosphoenolpyruvate carboxykinase
- Fructose 1,6-bisphosphatase
- Glucose 6-phosphatase

Describe glucose 6-phosphatase.
Glucose 6-Phosphatase
- Enzyme located in the lumen of the endoplasmic reticulum (ER).
- T1 transports Glucose 6-phosphate to ER.
- T2 transports Inorganic phosphate back into cytosol.
- T3 transports Glucose back to cytoplasm.

Describe glucose-6-phosphate dehydrogenase deficiency (G6PDD).
Glucose-6-Phosphate Dehydrogenase Deficiency (G6PDD)
- Prevalence:
- Most common genetic disease.
- ~400 million people have worldwide.
- ~7.5% allele frequency.
- Genotype/Mutation(s):
- X-linked, recessive.
- ~200 reported missense mutations.
- Phenotypic manifestations:

Describe glycogenesis.
Glycogenesis
- 3 Steps:
- Trapping and Activation of Glucose:
- Glucokinase/hexokinase in cytosol of hepatocytes and muscle cells catalyze phosphorylation of glucose to glucose-6-phosphate.
- Phosphoglucomutase then reversibly isomerizes glucose-6-phosphate to glucose-1-phosphate.
- Uridine diphosphate(UDP)-glucose pyrophosphorylase then transfers the glucose-1-phosphate to uridine triphosphate (UTP) which generates UDP-glucose (active form of glucose).
- Breakdown of pyrophosphate to Pi generates energy.
- Elongation of a glycogen primer:
- Preexisting glycogen
polymer serves as primer to
which glucose units are
added. - Glycogen synthase (rate
limiting enzyme). Catalyzes
transfer of glucose from
UDP-glucose to non-
reducing end of glycogen
chain. Forms α-1,4
glycosidic bonds between
glucose molecules.
- Preexisting glycogen
- Branching of glycogen chains:
- When glycogen chain reaches 11 residues, a fragment of the chain (about 7 residues long) is broken off at an α -1, 4 link and reattached elsewhere through an α
-1,6 link by glucosyl (4:6) transferase. - The new branch point must be at least 4 residues away from a preexisting branch.
- Branching increases solubility of glycogen and increases number of terminal non-reducing ends.
- Increases rate at which glycogen can be synthesized and degraded.
- When glycogen chain reaches 11 residues, a fragment of the chain (about 7 residues long) is broken off at an α -1, 4 link and reattached elsewhere through an α
- Trapping and Activation of Glucose:

Describe glycogenolysis.
Glycogenolysis
- Glycogen broken down to release glucose-1-phosphate.
- Glycogen remnant remodeled to permit further degradation.
- Glucose-1-phosphate converted to glucose-6-phosphate.
- Glycolysis
- Free glucose for release into blood stream.
- Pentose phosphate pathway – NADPH and ribose derivative.
- Four key enzymes:
- One to degrade glycogen (chain shortening).
- Two to remodel glycogen remnants.
- One to convert glycogen breakdown product suitable for further metabolism.

Describe glycolysis.
Glycolysis
- Involves a sequence of reactions that metabolizes:
- 1 molecule of glucose to 2 molecules of pyruvate and generates 2 ATP.
- Anaerobic process.
- Used to provide energy when requirements outpace O2 delivery.
- Pyruvate completely oxidized under aerobic conditions, generates much more ATP.
Describe gout.
Gout
- At high concentrations, urate crystallizes.
- Chronic elevated levels of urate in the blood (hyperuricemia) results in uric acid crystals collecting in joints = GOUT.
- Inflammation
- Arthritis
- Joint degeneration
- Many pathologies can produce gout symptoms, one is HPRT deficiency (Lesch-Nyhan Syndrome).

Describe how isozymes regulate.
Enzyme Regulation - Isozymes
- Can “mix and match” subunits.
- Catalyze same reaction but with different efficiencies.
- Tissue specificity - compartmentalized isozymes, different isozymes present in different tissues.
- Development - temporal expression of isozymes, different isozymes present at different time frames in development (e.g. HbF -> HbA).
Describe hydrolases.
Enzymes - Hydrolases
- Break a chemical bond by adding water across it (hydrolysis)

Describe integral membrane proteins.
Integral Membrane Proteins
- Interact extensively with the hydrocarbon chains of membrane lipids, and can be released only by agents that compete for these nonpolar interactions.
- Transmembrane regions of proteins have hydrophobic AAs displayed on their surfaces.
- α-helices are most common structure for transmembrane region, but can be beta sheet as in bacterial porin.
- Cotranslational insertion - feed into translocation channel directly from ribosome.

Describe irreversible inhibitors.
Inhibition - Irreversible
- Inhibition is permanent.
- Form covalent interactions.

Describe isomerases.
Enzymes - Isomerases
- Rearrange order of atoms in a molecule (isomerization).

Compare isozymes and enzyme level control in enzyme regulation.
Isozymes vs Enzyme Level Control

Describe ketone bodies.
Ketone Bodies
- Water-Soluble and Acidic Compounds:
- Acetoacetate
- β-Hydroxybutyrate
- Acetone
- Produced in Liver Only:
- Provide energy for peripheral tissues during fasting & brain during starvation!!!!
- Fasting/Starvation = Excessive β-oxidation of FAs –> ↑[Acetyl-CoA].

Describe ligases.
Enzymes - Ligases
- Paste two pieces together (make a chemical bond), uses ATP.
- Activated carriers:
- +/- aldehyde group (-COH):
- TPP
- +/- acyl group (-COR):
- CoASH
- Lipoamide
- +/- CO2 group:
- Biotin
- +/- aldehyde group (-COH):

Describe lyases.
Enzymes - Lyases
- Break chemical bond without using water.

Describe lysosomal glycogenolysis.
Glycogenolysis - Lysosomal
- Small amount of glycogenolysis occurs in lysosomes.
- In Pompe disease lysosomes become engorged with glycogen because they lack α-1,6-glucosidase, a hydrolytic enzyme confined to these organelles.

Describe malonyl-CoA.
Malonyl CoA
- Substrate for Fatty Acid Synthase (FAS).
- Regulator – inhibits carnitine acyltransferase (rate limiting step in FA degradation).
- Prevents FA synthesis and degradation from occurring simultaneously.
Describe membrane proteins.
Membrane Proteins
- Constitute ~30% of the proteome.
- Receive external signals.
- Transmit signals into cytoplasm.
- Transmit signals to another cell.
- Allow solutes through the membrane.
- Help to determine membrane thickness and rigidity.

Describe Mode 1 of the PPP.
Pentose Phosphate Pathway - Mode 1
- Utilized when the needs of ribose 5-phosphate >> NADPH.
- Produces primarily ribose 5-phosphate.
- Example:
- Rapidly dividing cells that need ribose 5-phosphate for synthesis of DNA precursors.

Describe Mode 2 of the PPP.
Pentose Phosphate Pathway - Mode 2
- Utilized when the needs for NADPH and ribose 5-phosphate are equal.
- Only proceeds through the oxidative phase.

Describe Mode 3 of the PPP.
Pentose Phosphate Pathway - Mode 3
- Utilized when the need for NADPH >> ribose 5-phosphate.
- Produces primarily NADPH.
- Example:
- Cells undergoing FA biosynthesis (adipose).

Describe Mode 4 in the PPP.
Pentose Phosphate Pathway - Mode 4
- Utilized when both NADPH and ATP are needed.
- Produces NADPH and ATP.

Describe negatively charged amino acids.
Amino Acids - Negatively Charged
- Can accept a proton.

Describe noncompetitive inhibition.
Inhibition - Noncompetitive
- Inhibitor binds at allosteric site.
- Can bind before or after S binds to active site.
- Lineweaver-Burk plots for rate with and without inhibitor, cross at x-intercept.
- νmax is variable.
- Km is constant.

Describe nucleic acid growth.
Nucleic Acids - Growth
- Biologically, always grows in the 5’ -> 3’ direction.
- In vitro oligonucleotide synthesis proceeds 3’ -> 5’.

Describe oxidative phosphorylation.
Oxidative Phosphorylation
- TCA cycle generates NADH and FADH2.
- In OxPhos these high energy electrons flow through 4 protein complexes called the electron transfer chain (ETC).
- Electrons reduce molecular O2 to water.
- Three of the complexes pump protons from matrix to intermembrane space.
- Protons return to matrix by flowing through another complex called ATP synthase.
- ATP synthesis.
- A successful OxPhos must accomplish the following key goals:
- Transfer electrons from NADH and FADH2 –> O2.
- Establish a proton gradient across the inner mitochondrial membrane H+.
- Synthesize ATP.

Describe oxidoreductases.
Enzymes - Oxidoreductases
- Move electrons (redox reactions)
- Activated carriers: NADH, NADPH, FADH2, FMNH2.

Describe P-type ATPases.
P-Type ATPases
- Use ATP.
- Couple phosphorylation and conformational changes to pump ions across membranes.
- 2 principal conformational states:
- Binding sites open to one side of membrane and binding sites open to other side of membrane.
- Interconversion between conformational states must be coupled with ATP hydrolysis.
- Example - SERCA:
- Pumps Ca2+ from cytosol into SR in skeletal muscle.
- Can bind 2 Ca2+, each coordinated with 7 O atoms.
- 3 domains:
- A domain - serves as actuator, linking changes in N and P domains to the transmembrane part of the enzyme.
- P domain - accepts phosphoryl group.
- N domain - binds the ATP nucleotide
- Catalytic cycle:
- With the release of phosphate, the interactions stabilizing the E2 conformation are lost, and the enzyme everts to the E1 conformation.
- The phosphorylaspartate residue is hydrolyzed to release inorganic phosphate.
- Upon ADP release, the enzyme again changes its overall conformation, including the membrane domain this time. This new conformation is referred to as E2 or E2-P in its phosphorylated form. The process of interconverting the E1 and E2 conformations is sometimes referred to as eversion.
- The catalytic cycle begins with the enzyme in its unphosphorylated state with two calcium ions bound. We will refer to the overall enzyme conformation in this state as E1 with Ca2+ bound, it is E1 -(Ca2+)2. In this conformation, SERCA can bind calcium ions only on the cytoplasmic side of the membrane.
- In the E1 conformation, the enzyme can bind ATP. The N, P, and A domains undergo substantial rearrangement as they close around the bound ATP, but there is no substantial conformational change in the transmembrane domain. The calcium ions are now trapped inside the enzyme.
- The phosphoryl group is then transferred from ATP to Asp 351.
Describe peripheral membrane proteins.
Peripheral Membrane Proteins
- Bound to membrane primarily by electrostatic and H-bond interactions with the head groups of lipids.
- May be bound to the surfaces of integral proteins.
- Some are anchored to the lipid bilayer by a covalently attached hydrophobic chain, such as a fatty acid.

Describe phase 1 of FA synthesis.
Fatty Acid Synthesis - Phase 1
- Transport of Acetyl CoA from mitochondria to cytoplasm.
- Step 1 - Condensation of Acetyl CoA with oxaloacetate (OAA) to form citrate. Catalyzed by citrate synthase.
- Step 2 - Transport of citrate from mitochondria to cytosol. Via a citrate transporter.
- Step 3 - Citrate converted back to Acetyl CoA and OAA. Catalyzed by citrate lyase** **(check out its regulation in the schematic!!!).
- Acetyl CoA used for FA synthesis in cytoplasm.
- Step 4 - OAA reduced to malate by malate dehydrogenase.
- Regeneration of OAA - Two mechanisms:
- Step 5 - Malate transported into mitochondria via malate-α-ketoglutarate transporter and oxidized to OAA by malate dehydrogenase.
- Step 6 - Cytosolic malate converted to pyruvate by malic enzyme. Pyruvate transported to mitochondria via pyruvate transporter and carboxylated to OAA by pyruvate carboxylase.

Describe phase I of fatty acid breakdown.
Fatty Acid Breakdown - Activation

Describe phase II of FA synthesis.
Fatty Acid Synthesis - Phase II
- Acetyl-CoA (2 carbon) is converted to Malonyl CoA (3 carbon) by carboxylation, catalyzed by Acetyl CoA Carboxylase (ACC).
- Rate limiting step of fatty acid biosynthesis.
- ACC adds a CO2 to Acetyl-CoA.
- Uses ATP (for energy) and Biotin as co-factor.
- Exists in dimeric (inactive) or polymeric (active) forms (Check out the regulation of ACC in schematic!!!).

Describe phase II of fatty acid breakdown.
Fatty Acid Breakdown - Phase II (β-Oxidation)
- Acyl CoA dehydrogenase (ACAD) - oxidizes the β carbon to produce FADH2 and trans-enoyl-CoA. FADH2 enters ETC to generate 2 ATP.
- Enoyl CoA hydratase saturates the alkene with water to form β hydroxy acyl CoA.
- β hydroxy acyl CoA dehydrogenase oxidizes the carbon to form ketoacyl CoA and NADH. NADH enters ETC to generate 3 ATP.
- Acyl CoA acyl transferase or ketothiolase attaches Sulfur of CoA to ketone formed from cleavage of acetyl CoA from fatty acyl chain which is shortened by 2C.
- Generate:
- FADH2 (delivers electrons to CoQ/ubiquinone of ETC).
- NADH (delivers electrons to Complex I of ETC).
- Acetyl CoA (enters TCA cycle).
- This group of 4 reactions repeated until FA broken down to acetyl CoA.