62. (Nelson) - Anemia and Hyperbilrubinemia Flashcards
Embryonic hematopoiesis
Begins…
Occurs where in midgestation and last trimester?
Hemoglobin concentration increases from… to…
What is the response of fetal hypoxia and anemia?
Embryonic hematopoiesis begins by the 20th day of gestation
and is evidenced as blood islands in the yolk sac.
In midgestation,
erythropoiesis occurs in the liver and spleen; the bone marrow
becomes the predominant site in the last trimester.
Hemoglobin
concentration increases from 8-10 g/dL at 12 weeks to 16.5-18 g/
dL at 40 weeks.
Fetal red blood cell (RBC) production is
responsive to erythropoietin; the concentration of this hormone
increases with fetal hypoxia and anemia.
- Hemoglobin levels after birth (term and preterm)
- Fetal and neonatal RBCs life span and mean corpuscular volume (MCV)
- Fetal hemoglobin (Hbg F)
- Synthesis
- Composition
- Percentage at term birth
- Levels decline to adult levels by what age?
After birth, hemoglobin levels increase transiently at 6-12 hours, then decline to 11-12 g/dL at 3-6 months.
A premature infant (<32 weeks’ gestational age) has a lower hemoglobin concentration and a more rapid postnatal decline of hemoglobin level, which achieves a nadir 1-2 months after birth.
Fetal and neonatal RBCs have a shorter life span (70-90 days) and a higher mean corpuscular volume (110-120 fL) than adult cells.
In the fetus, hemoglobin synthesis in the last two trimesters of
pregnancy produces fetal hemoglobin (hemoglobin F), composed
of two alpha chains and two gamma chains. Immediately before
term, the infant begins to synthesize beta-hemoglobin chains;
the term infant should have some adult hemoglobin (two alpha
chains and two beta chains). Fetal hemoglobin represents 60-90%
of hemoglobin at term birth.The levels decline to adult levels
of less than 5% by 4 months of age.
- Blood volume in term and preterm infant
- Blood volume in placenta and umbilical vessels
- Effects of Delayed/Early clamping of umbilical cord
For a term infant, blood volume is 72-93 mL/kg, and for a
preterm infant, blood volume is 90-100 mL/kg.
The placenta
and umbilical vessels contain approximately 20-30 mL/kg of
additional blood that can increase neonatal blood volume and
hemoglobin levels transiently for the first 3 days of life if clamping
or milking (stripping) of the umbilical cord is delayed at birth.
-
Delayed clamping usually has no adverse effects but
may increase the risk of polycythemia and jaundice. - Early clamping may lead to anemia, a cardiac murmur, poor peripheral perfusion, and less tachypnea.
Hydrostatic pressure affects blood transfer
between the placenta and the infant at birth. An undesired
fetal-to-placental transfusion occurs if the infant is situated
above the level of the placenta.
Decreased Red Blood Cell Production
Appears at birth with…
Potential causes of neonatal decreased RBC production include…
Anemia caused by decreased production of RBCs appears at
birth with pallor, a low reticulocyte count, and absence of
erythroid precursors in the bone marrow.
Potential causes of neonatal decreased RBC production include:
- Bone marrow failure syndromes (congenital RBC aplasia [Blackfan-Diamond anemia])
- Infection (congenital viral infections [parvovirus, rubella], acquired bacterial or viral sepsis)
- Congenital leukemia
Increased Red Blood Cell Destruction
ABO blood group incompatibility with neonatal hemolysis
develops only if…
Such as in…
ABO incompatibility with sensitization does not cause…
But it may cause… manifested as…
In contrast to Rh disease, ABO
hemolytic disease does not become…
ABO incompatibility has become the most common cause of…
ABO blood group incompatibility with neonatal hemolysis
develops only if the mother has IgG antibodies from a
previous exposure to A or B antigens. These IgG antibodies
cross the placenta by active transport and affect the fetus or
newborn. Sensitization of the mother to fetal antigens may
have occurred by previous transfusions or by conditions of
pregnancy that result in transfer of fetal erythrocytes into
the maternal circulation, such as first-trimester abortion,
ectopic pregnancy, amniocentesis, manual extraction of the
placenta, version (external or internal) procedures, or normal
pregnancy.
ABO incompatibility with sensitization usually does not
cause fetal disease other than extremely mild anemia.Itmay
produce hemolytic disease of the newborn, which is manifested
as significant anemia and hyperbilirubinemia.
Because many mothers who have blood group O have IgG antibodies to A and B before pregnancy, the firstborn infant of A or B blood type may be affected. In contrast to Rh disease, ABO
hemolytic disease does not become more severe with subsequent
pregnancies. Hemolysis with ABO incompatibility is less severe
than hemolysis in Rh-sensitized pregnancy, either because the
anti-A or anti-B antibody may bind to nonerythrocytic cells
that contain A or B antigen or because fetal erythrocytes have
fewer A or B antigenic determinants than they have Rh sites.
With the declining incidence of Rh hemolytic disease, ABO
incompatibility has become the most common cause of neonatal
hyperbilirubinemia requiring therapy, currently accounting
for approximately 20% of clinically significant jaundice in the
newborn.
Erythroblastosis fetalis
Erythroblastosis fetalis is caused by…
When does Erythroblastosis fetalis occur?
When is there an antibody response in the mother?
How do you diagnose it?
How does it affect the first newborn? (Signs/Symptoms)
How does it result in subsequent pregnancies and why?
What signs/symptoms occur in these subsequent pregnancies?
Erythroblastosis fetalis classically is caused by Rh blood
group incompatibility. Most Rh-negative women have no anti-Rh
antibodies at the time of their first pregnancy.
The Rh antigen
system consists of five antigens: C, D, E, c, and e; the d type
is not antigenic. In most Rh-sensitized cases, the D antigen of
the fetus sensitizes the Rh-negative (d) mother, resulting in
IgG antibody production during the first pregnancy.
Because
most mothers are not sensitized to Rh antigens at the start of
pregnancy, Rh erythroblastosis fetalis is usually a disease of the second and subsequent pregnancies.
The first affected pregnancy
results in an antibody response in the mother, which may be
detected during antenatal screening with the Coombs test and
determined to be anti-D antibody.
The first affected newborn
may show no serious fetal disease and may manifest hemolytic
disease of the newborn only by the development of anemia
and hyperbilirubinemia.
Subsequent pregnancies result in an
increasing severity of response because of an earlier onset of
hemolysis in utero.
Fetal anemia, heart failure, elevated venous
pressure, portal vein obstruction, and hypoalbuminemia result
in fetal hydrops, which is characterized by ascites, pleural and
pericardial effusions, and anasarca (see Chapter 60). The risk
of fetal death is high.
Management of pregnancy complicated by Rh sensitization
Management depends on…
How is severity of the hemolysis assessed?
The management of a pregnancy complicated by Rh sensitization
depends on the severity of hemolysis, its effects on
the fetus, and the maturity of the fetus at the time it becomes
affected.
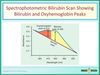
The severity of the hemolysis can be assessed by the
quantity of bilirubin transferred from the fetus to the amniotic
fluid, quantified by spectrophotometric analysis of the optical
density (at 450 nm) of amniotic fluid.
Prevention of sensitization of the mother carrying an Rh positive
fetus
When is it possible to treat the mother? What is the treatment and the dose? Which antigen(s) is the treatment effective in preventing sensitization?
Prevention of sensitization of the mother carrying an Rh positive
fetus is possible by treating the mother during gestation
(>28 weeks’ gestational age) and within 72 hours after birth
with anti-Rh-positive immune globulin (RhoGAM).
The dose
of RhoGAM (300 μg) is based on the ability of this amount of anti-Rh-positive antibody to bind all the possible fetal Rh positive
erythrocytes entering the maternal circulation during
the fetal-to-maternal transfusion at birth (approximately 30 mL).
RhoGAM may bind Rh-positive fetal erythrocytes or interfere
with maternal anti-Rh-positive antibody production by another,
unknown mechanism. RhoGAM is effective only in preventing
sensitization to the D antigen.
Blood loss
Causes of Acute blood loss
Acute blood loos is characterized by…
How are the hemoglobin levels affected?
Causes of Chronic blood loss
Chronic blood loss present with…
Anemia from blood loss at birth is manifested by two patterns
of presentation, depending on the rapidity of blood loss. Acute
blood lossafterfetal-maternal hemorrhage, rupture of the
umbilical cord, placenta previa, or internal hemorrhage (hepatic
or splenic hematoma; retroperitoneal)ischaracterized by pallor,
diminished peripheral pulses, and shock. There are no signs
of extramedullary hematopoiesis and no hepatosplenomegaly.
The hemoglobin content and serum iron levels initially are
normal, but the hemoglobin levels decline during the subsequent
24 hours.
Newborns with chronic blood loss caused by chronic
fetal-maternal hemorrhage or a twin-to-twin transfusion present
with marked pallor, heart failure, hepatosplenomegaly with or
without hydrops, a low hemoglobin level at birth, a hypochromic
microcytic blood smear, and decreased serum iron stores.
Fetal-maternal bleeding occurs in 50-75% of all pregnancies,
with fetal blood losses ranging from 1 to 50 mL; most blood
losses are 1 mL or less, 1 in 400 are approximately 30 mL, and
1 in 2,000 are approximately 100 mL.
Diagnosis of fetal-maternal hemorrhage
Diagnosis is confirmed by…
What is observed in the test?
What may cause a false-positive result?
What may cause a false-negative result?
The diagnosis of fetal-maternal hemorrhage is confirmed
by the Kleihauer-Betke acid elution test.
Pink fetal RBCs
are observed and counted in the mother’s peripheral blood
smear because fetal hemoglobin is resistant to acid elution;
adult hemoglobin is eluted, leaving discolored maternal cells
(patients with sickle cell anemia or hereditary persistence of
fetal hemoglobin may have a false-positive result, andABO
incompatibility may produce a false-negative result).
Diagnosis
What laboratory evaluation required for an infant suspected of hemolysis?
What findings are expected in isoimmune hemolysis?
What can be observed commonly in ABO incompatibility?
What is needed to determine the responsible antigen and antibody in an immunologically mediated hemolysis?
What other causes of nonimmune hemolysis must be considered?
What may be indicated when nonimmune hemolysis is suspected?
What may present in internal hemorrhage?
What may present in hemolytic diseases?
Because hydrops, anemia, or jaundice is secondary to many
diverse causes of hemolysis, a laboratory evaluation is needed
in all patients with suspected hemolysis. A complete blood
count, blood smear, reticulocyte count, blood type, and direct
Coombs test (to determine the presence of antibody-coated
RBCs) should be performed in the initial evaluation of all
infants with hemolysis.
Reduced hemoglobin levels, reticulocytosis,
and a blood smear characterized by polychromasia
and anisocytosis are expected with isoimmune hemolysis.
Spherocytes commonly are observed in ABO incompatibility.
The determination of the blood type and the Coombs test identify the
responsible antigen and antibody in immunologically mediated hemolysis.
In the absence of a positive Coombs test and blood group
differences between the mother and fetus, other causes of
nonimmune hemolysis must be considered. RBC enzyme
assays, hemoglobin electrophoresis, or RBC membrane tests
(osmotic fragility, spectrin assay) should be performed. Internal
hemorrhage also may be associated with anemia, reticulocytosis,
and jaundice when the hemorrhage reabsorbs; ultrasound
evaluation of the brain, liver, spleen, or adrenal gland may be
indicated when nonimmune hemolysis is suspected.
Shock is
more typical in patients with internal hemorrhage, whereas
in hemolytic diseases, heart failure may be seen with severe
anemia. Evaluation of a possible fetal-maternal hemorrhage
should include the Kleihauer-Betke test.
Treatment of Blood loss
Treatment of symptomatic neonatal anemia
What nonblood products may be necessary for resusciation of acute volume loss?
How much packed RBCs is sufficient to correct anemia and any remaining blood volume deficit?
The treatment of symptomatic neonatal anemia is transfusion
of cross-matched packed RBCs.
If immune hemolysis is
present, the cells to be transfused must be cross-matched against
maternal and neonatal plasma.
Acute volume loss may necessitate
resuscitation with nonblood products, such as saline if blood is
not available; packed RBCs can be given subsequently.
To correct
anemia and any remaining blood volume deficit, 10-15 mL/kg of
packed RBCs should be sufficient.
Cytomegalovirus-seronegative
blood should be given to cytomegalovirus-seronegative infants,
and all blood products should be irradiated to reduce the risk
of graft-versus-host disease; blood should be screened for HIV,
hepatitis B and C, and syphilis.
Recombinant erythropoietin
may improve the hematocrit in infants with a hyporegenerative
anemia after in utero transfusion.
[MAY SKIP THIS]
Physiology/Pathophysiology of Bilirubin
How is Bilirubin produced?
How much Bilirubin is there in a newborn compared to an adult
and why?
Bilirubin produced after hemoglobin catabolism is…
Indirect-reacting, unconjugated bilirubin is toxic to…
Unconjugated bilirubin binds to…
What happens if the binding sites become saturated?
What can displace bilirubin from its binding site?
Which enzyme conjugates bilirubin to bilirubin diglucuronide?
What are the properties of bilirubin diglucuronide?
How are concentrations of the enzyme in newborns?
Which test gives direct reaction to conjugated bilirubin?
Where is most conjugated bilirubin excreted?
What do bacteria in the neonatal inestine do?
Bilirubin is produced by the catabolism of hemoglobin in
the reticuloendothelial system. The tetrapyrrole ring of heme
is cleaved by heme oxygenase to form equivalent quantities of
biliverdin and carbon monoxide. Because no other biologic
source of carbon monoxide exists, the excretion of this gas
is stoichiometrically identical to the production of bilirubin.
Biliverdin is converted to bilirubin by biliverdin reductase. One
gram of hemoglobin produces 35 mg of bilirubin. Sources of
bilirubin other than circulating hemoglobin represent 20% of
bilirubin production; these sources include inefficient (shunt)
hemoglobin production and lysis of precursor cells in bone
marrow. Compared with adults, newborns have a twofold to
threefold greater rate of bilirubin production (6-10 mg/kg/24 hr
vs. 3 mg/kg/24 hr). This increased production is caused, in
part, by an increased RBC mass (higher hematocrit) and a
shortened erythrocyte life span of 70-90 days compared with
the 120-day erythrocyte life span in adults.
Bilirubin produced after hemoglobin catabolism is lipid
soluble and unconjugated and reacts as an indirect reagent in
the van den Bergh test.
Indirect-reacting, unconjugated bilirubin
is toxic to the central nervous system and is insoluble in water,
limiting its excretion.
Unconjugated bilirubin binds to albumin
on specific bilirubin binding sites; 1 g of albumin binds 8.5 mg
of bilirubin in a newborn.
If the binding sites become saturated
or if a competitive compound binds at the site, displacing bound
bilirubin, free bilirubin becomes available to enter the central
nervous system.
Organic acids such as free fatty acids and drugs
such as sulfisoxazole can displace bilirubin from its binding
site on albumin.
Bilirubin dissociates from albumin at the hepatocyte and
becomes bound to a cytoplasmic liver protein Y (ligandin).
Hepatic conjugation results in the production of bilirubin
diglucuronide, which is water soluble and capable of biliary
and renal excretion.Theenzyme glucuronosyltransferase
represents the rate-limiting step of bilirubin conjugation. The
concentrations of ligandin and glucuronosyltransferase are
lower in newborns, particularly in premature infants, than in
older children.
Conjugated bilirubin gives a direct reaction in the van den
Bergh test.
Most conjugated bilirubin is excreted through the
bile into the small intestine and eliminated in the stool. Some
bilirubin may undergo hydrolysis back to the unconjugated
fraction by intestinal glucuronidase, however, and may be
reabsorbed (enterohepatic recirculation).
In addition, bacteria
in the neonatal intestine convert bilirubin to urobilinogen
and stercobilinogen, which are excreted in urine and stool
and usually limit bilirubin reabsorption. Delayed passage of
meconium, which contains bilirubin, also may contribute to
the enterohepatic recirculation of bilirubin.
Bilirubin is produced in utero by the normal fetus and by the
fetus affected by erythroblastosis fetalis. Indirect, unconjugated,
lipid-soluble fetal bilirubin is transferred across the placenta
and becomes conjugated by maternal hepatic enzymes. The
placenta is impermeable to conjugated water-soluble bilirubin.
Fetal bilirubin levels become only mildly elevated in the presence
of severe hemolysis, but may increase when hemolysis produces
fetal hepatic inspissated bile stasis and conjugated hyperbilirubinemia.
Maternal indirect (but not direct) hyperbilirubinemia
also may increase fetal bilirubin levels.
Physiological jaundice
What causes physiological jaundice?
What is the peak indirect-reacting bilirubin levels in infants?
What is the peak indirect-reacting bilirubin levels in premature infants?
Peak indirect-reacting bilirubin in breast milk-fed vs. formula-fed infants?
What are the criterias for when jaundice is unphysiological/pathological?
Physiological jaundice is a common cause of hyperbilirubinemia
among newborns. It is a diagnosis of exclusion, made after
careful evaluation has ruled out more serious causes of jaundice,
such as hemolysis, infection, and metabolic diseases. Physiological
jaundice is the result of many factors that are normal
physiological characteristics of newborns: increased bilirubin
production resulting from an increased RBC mass, shortened
RBC life span, and hepatic immaturity of ligandin and glucuronosyltransferase.
Physiological jaundice may be exaggerated
among infants of Greek and Asian ancestry.
The clinical pattern of physiological jaundice in term infants
includes a peak indirect-reacting bilirubin level of no more than
12 mg/dL on day 3 of life. In premature infants, the peak is
higher (15 mg/dL) and occurs later (fifth day).
The peak level of
indirect bilirubin during physiological jaundice may be higher in
breast milk–fed infants than in formula-fed infants (15-17 mg/
dL versus 12 mg/dL). This higher level may be partly a result of
the decreased fluid intake of infants fed breast milk.
Jaundice is unphysiological or pathological
(1) if it is clinically evident on the first day of life,
(2) if the bilirubin level increases more than 0.5 mg/dL/hr,
(3) if the peak bilirubin is greater than 13 mg/dL in term infants,
(4) if the direct bilirubin fraction is greater than 1.5 mg/dL,
(5) or if hepatosplenomegaly and anemia are present.
Crigler-Najjar syndrome & Gilbert disease
What characterizes Crigler-Najjar syndrome?
Difference between type I and type II?
What is Gilbert’s syndrome caused by?
What does Gilbert’s syndrome result in?
Crigler-Najjar syndrome is a serious, rare, autosomal
recessive, permanent deficiency of glucuronosyltransferase that
results in severe indirect hyperbilirubinemia.
Type II responds to enzyme induction by phenobarbital, producing an increase in enzyme activity and a reduction of bilirubin levels. Type I
does not respond to phenobarbital and manifests as persistent
indirect hyperbilirubinemia, often leading to kernicterus.
Gilbert disease is caused by a mutation of the promoter region of
glucuronosyltransferase and results in a mild indirect hyperbilirubinemia.
In the presence of another icterogenic factor
(hemolysis), more severe jaundice may develop.